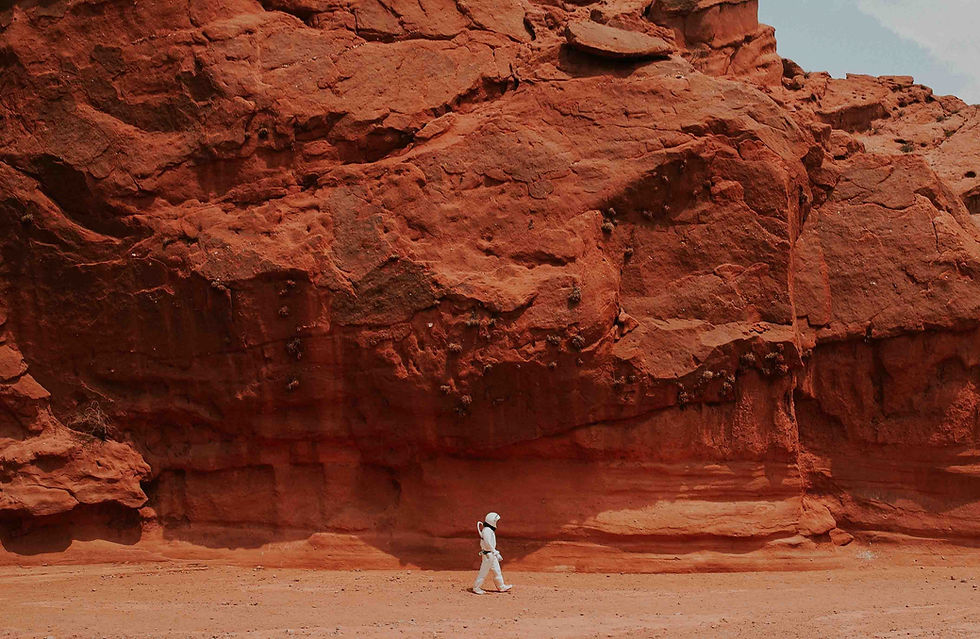
Why are rockets fundamental to our exploration of Mars? Rocket Engineering Ltd have been contributing to an article on Mars exploration, to be published in the Royal Aeronautical Society’s ‘Aerospace’ magazine and to appear in its ‘Aerospace Insight’ Blog later this summer. The article will, among other things, explain some of the challenges of getting to Mars. An excerpt is below: –
First, why explore Mars? Mars is one of Earth’s nearest neighbours, and has the most Earthlike surface conditions of any other body in the solar system. Life, if it does still exist on Mars is likely to be well hidden. But the fossil record it may have been left behind is the target of a fleet of missions launched this summer, as we entered our current energy efficient bi-annual ‘launch window’ for the Red Planet. Other reasons to explore, perhaps with human explorers is the potential to better understand how Mars might support longer term human habitation, perhaps for colonisation or even as a second home for the human race in the event of a catastrophe on our own planet.
So, why are rockets fundamental to our exploration of Mars? Rockets enable travel between the separate orbits of Earth and Mars, whose alignments restrict efficient or ‘minimum energy’ transfers to take place only every two years. Transfers to Mars also require about 1/3 as much energy as reaching Earth orbit from the ground (in technical terms, that’s a deltaV or total velocity change of around 3.5km/s). When a spacecraft reaches Mars, it can briefly fly past taking images or video, as did the earliest probes; or rendezvous and go into orbit around the planet. Rockets are further needed for rendezvous as a spacecraft travelling from Earth will be moving faster than Mars moves around the sun, when it arrives . On reaching Mars, spacecraft have the option of landing on the surface. Landing can be done either directly from the inbound Earth trajectory, known as hyperbolic entry, or after entering Mars orbit.
Each approach has its benefits and disadvantages, with the latter (landing from orbit) being more demanding from a total propulsion perspective . The deltaV required to decelerate from an orbit originating from Earth must then be added to the deltaV required to descend to the surface. What this comes down to is that for every 4kg of spacecraft, payload, or human crew placed into a low Earth orbit (which is already difficult), only 1kg of it will reach the surface of Mars, the difference being literally burnt in state-of-the-art propulsion systems to deliver it safely. And we’re not even talking about how return of payloads from Mars might be accomplished, which is some way off in the future.
Considering just the descent from a Mars orbit to a landing site, multiple independent systems such as heat shields, retro rockets parachutes and even airbags need to work in a sequence which is often pre-set on Earth and may be dependent for its success on the precise weather conditions on Mars. The latter is a subtle point but may have been the undoing of the UK’s small Mars lander Beagle 2, thought lost during touchdown in 2005, but identified intact from orbit in 2015. Beagle2 made a direct hyperbolic approach to land on Mars, having been transported most of the way by ESA’s Mars Express. It had no propulsion system and relied on a combination of heat shield, parachute and airbags, together with a radar altimeter and timers pre-programmed on Earth before departure. One theory for the failure was that Mars’ atmospheric density had changed between launch and arrival, such that it did not slow the lander sufficiently to allow the final airbag landing mechanism to soften its final fall after heatshield and airbags had been discarded.
Beagle2 mission entry descent & landing sequence (image credit: Beagle2 team)
Whether this is the case will probably never be known, but two fundamental lessons can be learned here:
The first is that landing on Mars is actually made more difficult than on many planetary bodies because it has an atmosphere. Mars’ atmosphere is neither thick enough to be particularly useful in descent (such as on Earth where the atmosphere plays a significant role in decelerating vehicles returning from orbit) , but is just thick enough to greatly increase the complexity of reaching the ground (unlike descent onto say the airless Moon where rocket thrusters can be used in a near automatic fashion to land softly). Mars atmosphere also varies significantly with season and also, sometimes, according to the weather. This provides a major driver for space missions to pause – in Mars orbit -to check the prevailing atmospheric conditions, first before making the last part of the journey to the surface. At least one mission, Russia’s Mars 3 was lost descending in 1971 through a dust storm to a site in the southern hemisphere. However -as pointed out earlier, the ability to drop into orbit round Mars as a ‘pit stop’ before a surface landing requires a propulsion system to decelerate.
The second point is that almost any mission to Mars requires a propulsion system, the requirements for which become progressively more demanding as the landing accuracy requirements and mission mass grows. Development of a number of propulsion systems specifically for exploring Mars has taken place across the world, with some notably examples being the Viking lander engine, the throttleable MR-80B used on Curiosity and the recently launched Perseverance, and in Europe the HTAE. Rocket Engineering worked on at the HTAE (also known as Leros 4), a European funded high thrust apogee rocket engine designed to support large missions performing orbit entry burns to planets such as Mars. See our ‘Projects’ page for an image of an early prototype of this engine being tested.
Artist’s impression of Skycrane delivering NASA’s Curiosity rover to Mars surface (image credit: NASA)
To finish our longest blog post to date, we can summarise that since October 1960, almost 50 missions, some comprising orbiters and landers have targeted Mars. Unfortunately only around 50% of landers have succeeded, with more successes in recent years particularly from US missions which have developed propulsion systems specifically for this job. Many attempts to land on Mars at low cost, ie avoiding the use of throttleable liquid engine propulsion systems have failed. You can find a complete list of Mars missions and links to their respective Wiki pages to find out more, at Wikipedia.
In the future ESA NASA and potentially other partners are contemplating a Mars Sample Return mission… This will be an extremely complex multi stage mission which at least initially will be broken into 2 parts, the first being to collect and cache samples (this is the easy part!), the second being to collect and return those samples to Earth. The second part, the actual sample return, will be the first time that an unmanned rocket system has been ignited and launched from a planet, then made its way back to Earth via a rendezvous with a return capsule waiting in Mars orbit. Surely a challenge for even the best rocket engineers!
You can find more information about Mars missions, landing on Mars, and the technologies used in recent large Mars landers at:
Rocket Engineering also recommend the excellent textbook ‘Expedition Mars’ by Martin J L Turner (Springer Praxis, 2004). Available from Amazon and all good book sellers.
Comments